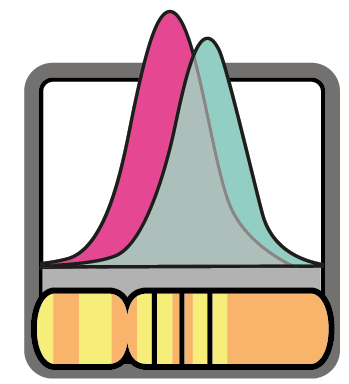
By Carol Morton
4 May 2021. Hundreds or thousands of small genetic variations can add up to raise – or lower – a person’s risk of different diseases, as many studies have suggested. But the multiple ways genetic risk scores are calculated and reported threaten their promising future in medicine.
To keep these potentially powerful tools on track to improve clinical care and public health, a pair of US and UK research teams have published new guidelines in the journal Nature. Called the Polygenic Risk Score Reporting Standards, the 22-item framework is designed to make genetic scores in studies more transparent, reproducible, and valid.
In a companion paper in Nature Genetics, the UK team presented the Polygenic Score (PGS) Catalog, an open database for study authors to show the math and metadata behind the published risk scores.
The catalog and reporting standards reflect a major transition in the role of genetic studies in human health. For the last 10 or 15 years, genome-wide association studies mostly have been used to probe the biology, with the genetic variants providing clues to molecular pathways in studies that help reveal the origin and progression of disease and possible druggable targets.
Now, in some diseases such as diabetes or prostate cancer, genomic information may have more direct value for medical care. In some diseases, polygenic scores are emerging as important risk factors, independent of family history and other standard clinical measures.
“We’re at the point where we can clearly differentiate individuals who are definitely high risk and those who are low risk” for some conditions, says Samuli Ripatti, deputy director of the Institute for Molecular Medicine in Finland (FIMM) at the University of Helsinki, who studies genetic risk and its use in disease prevention, and who was not involved in the papers.
“The methodologies and the scores are developing fast,” he says. “The usefulness of the work these teams have done is to set standards on how to report results in order to enable proper ways to benchmark algorithms and test how they work” in other populations. For example, he says, many PGSs seem to be robust in the European ancestry populations in which they were developed, but they may not be relevant to individuals of other ancestries.
In a February webinar, Ripatti described research by his team showing that polygenic risk scores can predict both earlier and later onset of breast cancer in Finnish women of European ancestry. The results suggest that some women may benefit from early screening, and others may need mammograms less often, rather than a one-size-fits-all approach to prevention and early detection. Ripatti and his colleagues are planning a pilot study in the fall of 2021 to test the addition of this information in the clinic.
For the past year, Ripatti’s group has contributed information from several studies to the PGS Catalog. “We put in the structured description of the work, as well as weights for individual genetic variants that go into the calculations,” he says. “Other researchers can take the weights and calculate using their own data and methods.”
Open Source and Standards
The pilot PGS Catalog was presented at the 2019 American Society of Human Genetics meeting. “The main goal of the Catalog is [to] provide an open source of polygenic [risk] scores so that others can better understand how they were developed and re-use them on their own datasets,” tweeted Sam Lambert, postdoctoral fellow in the University of Cambridge, UK, lab of Mike Inouye and first author of the catalog paper.
“Along the way, we noticed that the reporting of PGS studies was often varied, and scores were often not shared (~40% of the time),” Lambert tweeted. That launched a collaboration with a US team to update another best-practice model called the Genetic Risk Prediction Studies, published by an international working group in 2011.
The authors of the updated reporting standards paper counted more than 900 publications that mention polygenic risk scores (PRSs). Only a few diseases have mature PRSs with potential for clinical utility, such as breast cancer and cardiovascular disease. Health care systems are gearing up to deliver genetic risk information, and direct-to-consumer testing companies, such as 23andMe and others, already provide genetic risk scores to customers outside the traditional patient-provider framework.
“To move forward, it is vital for us to fully understand each other’s work,” tweeted Genevieve Wojcik, an epidemiologist at Johns Hopkins University, US, and senior author of the reporting paper, which she describes as “the minimal set of requirements for understanding the who, what, when, and how of PRS.”
The 22-item checklist answers such questions as “Why are you doing this study?” “Who are you studying?” “How did you make your score?” “How did you measure the performance of your score?” And finally, “What do you think this shows, and where should it go from here?” Wojcik tweeted.
The standards paper highlights the PGS Catalog as a venue for authors to deposit their polygenic scores and relevant metadata describing development and evaluation procedures and populations.
Ripatti suggests an additional use for the reporting standards and catalog. “Next time I’m reviewing a paper on polygenic risk scores for a journal, I’ll ask the authors if they have checked against these report standards,” he says. “In this way, we can help steer the field toward a more uniform way of describing things.”
References
The Polygenic Score Catalog as an open database for reproducibility and systematic evaluation.
Lambert SA, Gil L, Jupp S, Ritchie SC, Xu Y, Buniello A, McMahon A, Abraham G, Chapman M, Parkinson H, Danesh J, MacArthur JAL, Inouye M.
Nat Genet. 2021 Apr;53(4):420-425.
Improving reporting standards for polygenic scores in risk prediction studies.
Wand H, Lambert SA, Tamburro C, Iacocca MA, O'Sullivan JW, Sillari C, Kullo IJ, Rowley R, Dron JS, Brockman D, Venner E, McCarthy MI, Antoniou AC, Easton DF, Hegele RA, Khera AV, Chatterjee N, Kooperberg C, Edwards K, Vlessis K, Kinnear K, Danesh JN, Parkinson H, Ramos EM, Roberts MC, Ormond KE, Khoury MJ, Janssens ACJW, Goddard KAB, Kraft P, MacArthur JAL, Inouye M, Wojcik GL.
Nature. 2021 Mar;591(7849):211-219. Epub 2021 Mar 10.
Genetics of 35 blood and urine biomarkers in the UK Biobank.
Sinnott-Armstrong N, Tanigawa Y, Amar D, Mars N, Benner C, Aguirre M, Venkataraman GR, Wainberg M, Ollila HM, Kiiskinen T, Havulinna AS, Pirruccello JP, Qian J, Shcherbina A; FinnGen, Rodriguez F, Assimes TL, Agarwala V, Tibshirani R, Hastie T, Ripatti S, Pritchard JK, Daly MJ, Rivas MA.
Nat Genet. 2021 Feb;53(2):185-194.
The role of polygenic risk and susceptibility genes in breast cancer over the course of life.
Mars N, Widén E, Kerminen S, Meretoja T, Pirinen M, Della Briotta Parolo P, Palta P; FinnGen, Palotie A, Kaprio J, Joensuu H, Daly M, Ripatti S.
Nat Commun. 2020 Dec 14;11(1):6383.
Polygenic and clinical risk scores and their impact on age at onset and prediction of cardiometabolic diseases and common cancers.
Mars N, Koskela JT, Ripatti P, Kiiskinen TTJ, Havulinna AS, Lindbohm JV, Ahola-Olli A, Kurki M, Karjalainen J, Palta P; FinnGen, Neale BM, Daly M, Salomaa V, Palotie A, Widén E, Ripatti S.
Nat Med. 2020 Apr;26(4):549-557.
Genomic prediction of coronary heart disease.
Abraham G, Havulinna AS, Bhalala OG, Byars SG, De Livera AM, Yetukuri L, Tikkanen E, Perola M, Schunkert H, Sijbrands EJ, Palotie A, Samani NJ, Salomaa V, Ripatti S, Inouye M.
Eur Heart J. 2016 Nov 14;37(43):3267-3278.
Genetic risk prediction and a 2-stage risk screening strategy for coronary heart disease.
Tikkanen E, Havulinna AS, Palotie A, Salomaa V, Ripatti S.
Arterioscler Thromb Vasc Biol. 2013 Sep;33(9):2261-6.